Particle physicist, Patrick Owen, explains recent evidence that suggests a revolution in our understanding of the universe.

When Dr. Patrick Owen and his colleagues saw the results of their most recent analysis of beauty quark decays, they knew they were looking at something that could have deep implications. Their reaction, preserved in a video of their Zoom call from March 2021, is characteristically understated. There are smiles, and the odd raised eyebrow, and muttered congratulations. One of the group leaders, Professor Nicola Serra, even offers a faint ‘Wow’.
But the muted response does not convey the magnitude of the evidence they had seen.
“It was exciting, absolutely. Very, very exciting,” Owen tells me. “I find unblinding results quite stressful. In this case it wasn't at all, because it was beautifully consistent with our previous readings. We cannot claim any discovery yet, but when I first saw the result I started to think this actually could be true.”
He is speaking from his office at the Physik-Institut of the University of Zurich where he is a Senior Researcher, working in the LHCb collaboration, one of the research groups analysing data from the Large Hadron Collider (LHC) housed at CERN in Geneva. He looks relaxed sitting back in a loose-fitting t-shirt, with diagrams of particle decays – Feynman diagrams to the initiated – scribbled over the whiteboard that is his backdrop. The appearance is so quintessentially ‘physics geek’ it almost looks set up.
“This is actually a new physics particle,” he says, pointing to one of the diagrams. “It’s called the Z' [pronounced zed-prime], and is one of the particles that could explain what we're seeing.”
To understand what Owen is describing, we need to consider the basics of what makes up matter. Most are familiar with the idea of atoms, but most GCSE students could tell you atoms themselves are composed of smaller particles.
“We are studying sub-atomic particles, so things that are even smaller than the atom,” Owen says. “There are the ones I think most people are familiar with: the electron, the proton and the neutron.
“But it turns out – and this was discovered about eighty years ago – there are additional, heavier copies. There is a heavier copy of the electron, called the muon, and then a super-heavy copy called the tauon. They only exist for microseconds or less, so these are exotic particles that you only produce in high energy collisions.
“For years we've built a theory about how they interact, called the Standard Model. It predicts that, apart from [being heavier or lighter], they all interact in exactly the same way.”
Owen and his colleagues study these interactions, and how the particles break up, or ‘decay’. Their recent results suggest that all might not be as the theory suggests.
“There seems to be a hint that the electron, the muon and the tauon are not just heavier copies, but they actually behave differently from each other,” he says. “And if this was confirmed, it would mean the Standard Model is wrong, and that would have profound implications.”
The intriguing result comes from measurements of the decay of one type of particle called the beauty quark, which breaks up to produce either electrons or their heavier cousins, muons. The Standard Model predicts that these two types of decay should happen at roughly the same rate; for every decay that yields electrons there will, on average, be one that produces muons.
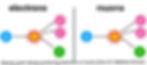
“It looks like electrons are being produced more often,” says Owen. “It's roughly fifteen per cent more likely to give an electron than a muon, but the Standard Model tells you it should be [almost] the same. This is why people are very excited.
“This implies a new interaction on top of the forces that we already know about, which treat electrons and muons the same. If we confirm this, it would mean a new fundamental force of nature, and new particles that were not predicted by the Standard Model. So, it would be a really big deal indeed.”
This new force would add to the four already known – gravity, electromagnetism, and the strong and weak nuclear forces – with the new evidence contradicting current thinking that the forces can only act differently on muons and electrons due to their different mass. Put simply, it would be like dropping a rock on Earth and measuring its acceleration, then dropping the same rock on the Moon and finding it fell much faster than the mass difference of the Earth and Moon suggests it should. Either there would have to be mass hiding somewhere, or some other, unknown effect would be at play, and we would need to re-think fundamentally the accepted physics of more than half a century.
“In my opinion it's a bigger paradigm shift than the Higgs boson,” says Owen of the particle discovered in 2012. “That was a brilliant discovery and fantastic effort, and a massive deal, but it was predicted to be part of the Standard Model. This is something that is not predicted.”
Owen stops short of claiming that a discovery would alter physics entirely, but he is excited at how significant a change it might be.
“It would be a revolution, because people at university would now be taught that there are five forces of nature, when we have been taught for the last fifty years that there were four. This really is rewriting textbooks stuff.”
Physicists are careful with their language, sticking to conventions that dictate when they can claim a discovery. Whenever we repeat the same type of measurement there will be some variation in the result. Interest is piqued only when results differ significantly from what would be predicted by ordinary chance. When tossing a coin, for example, we would expect heads and tails each to occur about half the time: a hundred tosses should be about 50/50 heads and tails. A result of 51/49 would be entirely normal, but what about 60/40 or 70/30 in favour of one or the other? Eventually, the results are so unlikely to have occurred by chance that we conclude there is probably something going on: a weighted coin, a biased experimenter, or in the case of particle physics, some new and mysterious force.
Owen explains: “You expect [measurements] on average would show decays producing electrons and muons are [almost] equally likely. The probability [that our results are a fluke] is about one in a thousand – or what we call ‘three-sigma’ – which is very unlikely statistically. Once you get above that [in particle physics], this is sufficient to claim ‘evidence for’ something. The ‘discovery’ threshold is ‘five-sigma’, or one in 3.5 million, which is where you can call the press and say you found something. We're in the exciting but not yet confirmed period.”
The group must now take further readings, and add new types of measurement to their analysis; measurements that could align with the Standard Model predictions and refute the existence of any new physics.
“Even if it is all true there might be bumps in the road as results fluctuate, so you can be in for a rough ride,” says Owen. “Of course, I'm not convinced yet, and it's our job to be sceptics of our own work. But I think we will find an answer within the next few years.”
The slow grind towards a conclusion is a product of the rigorous peer review cycles that are the foundation of scientific discovery. The LHC is due to begin harvesting data again in 2022 after a three-year pause, and at around five times its previous rate. There will be masses of data for the scientists to sift and analyse, and interpretations to review and criticise.
As Owen explains: “These analyses take a long time, even after all the data has been collected. There are petabytes of data and you're only interested in a small part. It’s like an ocean, and you have to find the particular thing that you're interested in, something that only happens one in a million times.”
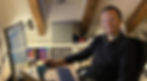
Day to day, Owen and his colleagues write computer code to extract relevant information from this ‘ocean’. Ideas are debated, often for as long as two or three years, and once everyone has agreed an analytical approach, they publish an ‘analysis note’, which in the case of the recent work ran to over six hundred pages. Fellow experts examine and criticise, and after further public presentations, questions and revisions, a group can be confident their analysis is sound. As Owen puts it, “This is why, when you do see something interesting, you can have the confidence to say, not yet that we have found something, but that we don't think we made a mistake here.”
And this brings us back to that Zoom call, and the excitement of the ‘unblinding’ of the results. Throughout the entire process of verifying their analysis, nobody can see the outcome: they are ‘blind’ to the result, a deliberate restriction that prevents bias.
“You're not allowed to look at the data, so you don't know the result until the very last minute,” says Owen. “You present to the whole collaboration, and say, ‘Here's the analysis, here is what we have done, we believe we are ready to unblind the results, do you agree?’ Once everyone is happy, you ask for formal permission to unblind: to look at the result for the first time. It is exciting when you finally get there.”
He insists it is not quite the showbiz-style big reveal that I imagine. Rather, they hold a meeting where graphs and probability calculations are shared. This is where the group saw results consistent with their previous observations: evidence for new physics.
“These bits of evidence often do tend to go away,” he says. “But to have seen a hint before, and then look at a completely independent dataset and also see it there, that really made me a bit of a believer.”
Whether their evidence becomes a discovery is one thing, but as we finish, I ask Owen what implications it could have outside the physics community.
“It’s difficult to foresee these things. When they first discovered the transistor, they had no idea it would lead to building a computer, for example. So, it’s difficult to see what applications there might be in the future.
“For the average person it would be cool to know a new force of nature had been discovered, and it is a huge deal for physics that there should be new particles to find. And maybe it can lead to answering some of the big questions: about dark matter and dark energy, matter and anti-matter.”
We might have to wait two or three years, but if Owen and his colleagues confirm a discovery, there surely will be more than a few quiet congratulations amid cautious excitement over Zoom. It would be a ‘Wow’ moment, indeed.
*
Dr. Matthew Kenzie of the University of Warwick explains the findings:
The group at the University of Zurich explain their work, including the moment of 'unblinding':